Increased working memory load activates superficial layers of the dorsolateral prefrontal cortex
The dorsolateral prefrontal cortex (dlPFC) is reliably engaged in working memory (WM), yet the lamina-specific circuitry underlying its function is not well understood. Evidence from non-human primate physiology suggests that the superficial layers of the dlPFC have recurrent connections that might underly delay period working memory maintenance, while deep layers are regarded as output layers with connections to premotor areas (Goldman-Rakic 1995, Miller et al. 2018). A previous human fMRI study (Finn et al., 2019) showed higher involvement of superficial layers for manipulation compared to mere remembering of verbal working memory stimuli. We hypothesized that such higher involvement would also be associated with increases in working memory load. Using laminar fMRI, we varied WM load and the requirement for a motor response in a delayed match-to-sample task and hypothesized that higher load will lead to preferential activation of the left dlPFC’s superficial layers, while action selection will activate the deep layers.
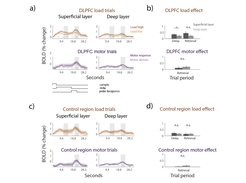
We acquired data from nine subjects using gradient echo based fMRI at 7T. Fig. 1a shows univariate trial time courses for superficial (left) and deep (right) layers in the dlPFC for load trials (high and low conditions; top plots) and motor trials (press and abstain conditions; bottom plots). The shaded area indicates standard error across participants. The gray transparent rectangles indicate the two trial periods of interest: the delay (11.3 s - 15.1 s) and the retrieval period (20.7 s - 24.5 s). We calculated a load effect (Fig. 1b) per subject by subtracting the low from the high load time course and averaging timepoints associated with the delay period (11.3 s - 15.1 s). The motor effect was calculated per subject by subtracting the abstain from the motor response time course and averaging timepoints in the retrieval period (20.7 s - 24.5 s). The star indicates a p<0.05 (Bonferroni corrected for multiple comparisons within an ROI). The error bars indicate standard error across participants. Figs. 1c and 1d are the same as Figs 1a and 1b respectively but for control regions.
In summary, Fig. 1 demonstrates that there was a univariate increase in superficial layer responses to higher load during the early delay period.
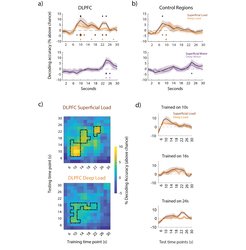
However, individual voxel responses within each layer of the ROI are not necessarily homogenous. To be more sensitive to such multivariate patterns, we trained a classifier (linear SVM) to differentiate between both load and motor conditions. In Fig. 2a the top plot shows the time course of decoding accuracy of high vs. low load conditions across time from the superficial (dark brown) and deep layers (light brown) layers of the dlPFC. The bottom plot shows the decoding accuracy of press vs. abstain motor conditions across time from the superficial (dark purple) and deep (light purple) layers of the dlPFC. Small, medium, and large diamonds indicate p<0.05, p<0.01, p<0.001 significance levels (not corrected for multiple comparisons across time), respectively. The diamonds below the time courses indicate above-chance decoding significance of superficial and deep layers (in their corresponding color). The black diamonds above the time courses indicate comparison between layers at a given time point. Fig. 2a is the same as Fig. 2b but for control regions. Fig 2c shows temporal cross-decoding of load from the superficial and deep layers of the dlPFC. The top and bottom plots indicate the superficial and the deep layers, respectively. A classifier trained on each timepoint was tested on itself and all other time points within a trial. The diagonal of the top and bottom plot corresponds to the classification accuracy of load in Fig. 2a. The demarcated points show all significant time points within a layer after cluster-permutation against null. Fig 2d shows time courses of decoding accuracy across the length of the trial when trained on the encoding (top plot; 10s), delay (middle plot; 16s), and retrieval (bottom plot; 24s) trial periods and tested on itself and all other time points. Columns at 10s, 16s, and 24s in Fig 2c correspond to the top, middle, and bottom time courses, respectively. The shaded area in the time courses indicates standard error across participants. In summary, Fig. 2 shows that there was a higher load decoding accuracy in superficial layers during the encoding, early delay, and retrieval periods. We were also able to decode the presence or absence of a motor response from both superficial and deep layers during the response period, yet no difference in layers was seen. Load and motor decoding results were stronger in the dlPFC compared to a set of left frontal control regions belonging to the cingulo-opercular network (COP). Finally, the temporal cross-decoding analysis indicated evolving response patterns in the superficial layers of the dlPFC. Our results support a more general role of superficial layers of the dlPFC in working memory maintenance with distinct population responses to encoding, delay and retrieval.